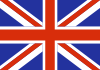
Temporary closure due to an electronic problem on the main telescope used for visits. We are waiting for parts to repair it.
image
We have discovered an extremely useful on-line resource which gives a good estimate of the darkness which can be expected on any night of the year, based on sunset, sunrise and twilight, as well as the Moon phase and when it rises and sets. More information is given in the ""Help and Details"" section below the calendar.
You can use it for any location, but in planning a visit to GersAstronomie you will need to enter our geographical location: 44 degrees latitude and 0.5 degrees longitude. Then enter any starting date you want and the number of days you want the calendar for.
The URL for ""Moon and Darkness Calendar"":
https://dersphere.github.io/moon-and-darkness-calendar/
This year, 2024, we have seen the aurora borealis (or "northern lights") at Le Bourdieu a couple of times. This is pretty unusual. They are caused when energetic particles and plasma, known as a coronal mass ejection, are launched from the Sun by a violent event called a flare, and intercept the Earth.
The Sun has a regular cycle of activity, with a period of about 11 years. This is most obviously visible in the number of sunspots on its face – we can safely observe sunspots and the arcing prominences (which look like flames at the edge of the Sun) with the solar telescope in our main observatory. At the minimum of the solar cycle there are rarely any visible, but now, at solar maximum, they are plentiful.
Early on 9 October 2024 there was a large flare, classified as X1.8 which was perfectly placed to launch a coronal mass ejection straight at Earth!
Sure enough, in the evening of 10 October we were alerted by friends spread over northern Europe that an auroral display was in progress. We had missed the superb aurora of 10 May 2024 (though our observatory all-sky camera recorded it in black and white), so were anxious to see this one.
It wasn't very promising: there was cloud to the north as well as moonlight. There was nothing visible to the eye, even after allowing time for adaptation to the dark, but we knew the iPhone camera is able to capture faint objects so tried it. Well, there is a red aurora there, peeping through cloud, but it's not very impressive.
The most common auroral colour is green, which is caused by charged particles colliding with oxygen molecules between 100 and 300 km above the surface. Green auroras are often seen in combination with red ones above them, which are caused by charged particles interacting with free oxygen atoms higher up, at 300 to 400 km.
So, this red aurora is the upper part of a display which would be green lower down, but below our horizon here, so far south.
The eye is much more sensitive to green and, just a few weeks before, we could see this in dramatic fashion. At the end of September we were at a scientific meeting in Reykjavik, Iceland, where aurora are visible practically every clear night. Sure enough, we could see them from the hotel's roof terrace in downtown Reykjavik, easily visible to the unaided eye (even with a bright Moon) and dancing around. No special solar activity was needed this time: Iceland is sufficiently far north that auroras are visible most nights.
There's no red visible in this example, but other pictures we took do show red traces, and others at the meeting, who had ventured out of town, saw the red component too.
The Sun will remain active, at its maximum of the current solar for many months yet, so we have great hopes of seeing more auroras at Le Bourdieu !
For several years, Richard has been taking photographs of nebulae, galaxies, stars, deep space objects a few hundred light years away with his state-of-the-art telescopes and cameras.
Finally, he gave in to the request of friends; the summer exhibition of some of his astro-photographs was inaugurated on July 3, 2024 during a warm opening at La Collégiale de La Romieu, one of the most beautiful villages in France. The exhibition of 33 celestial photos, all taken at Le Bourdieu observatory, ends on October 1. But this is only the beginning.